Project links:
- M.S. Thesis: Deep seawater incursion and high-temperature amphibole growth along cross-cutting detachment faults at the Southwest Indian Ridge
- Wyoming State Geological Survey: Geological Map Series of the Phantom Lake Quadrangle
- Undergraduate Honors Thesis: 3D-Modeling and Magnetic (AMS) Analysis of Mesozoic Diabase Dikes in North Carolina
1. Deep seawater incursion and high-temperature amphibole growth along cross-cutting detachment faults at the Southwest Indian Ridge
Master’s Thesis Research, University of Wyoming (2019 – Present)
Daniel J. Colwell1, Michael J. Cheadle1, Barbara E. John1, Susan M. Swapp1, Daniele Brunelli2, Henry J.B. Dick3, and Benjamin M. Urann1,3
1University of Wyoming, 2University of Modena and Reggio Emilia, 3Woods Hole Oceanographic Institution
Hydrothermal circulation at mid-ocean ridges (MORs) is an essential part of seafloor geochemical cycling that drives mineralization, nourishes ecosystems, facilitates deformation of the lithosphere, and influences the initiation and slip of normal faults. Plastically deformed peridotites exhumed by gently dipping, large-offset normal faults at the Southwest Indian Ridge (SWIR) contain evidence of the depth and conditions of fluid-rock reactions and plastic deformation below one of the slowest spreading MORs on Earth. To characterize this system, I analyzed the microstructure and chemical composition of 31 amphibole-bearing peridotite mylonite samples dredged from the ~43–45 °E segment of the SWIR (Fig. 1), where there is little or no magmatism and faulting dominates plate spreading. Recent seismic studies elsewhere along the SWIR predict a 13–20 km thick elastic lithosphere and, consequently, a deep brittle-plastic transition, suggesting that normal faults transport peridotite mylonites from >13 km depth to the surface.
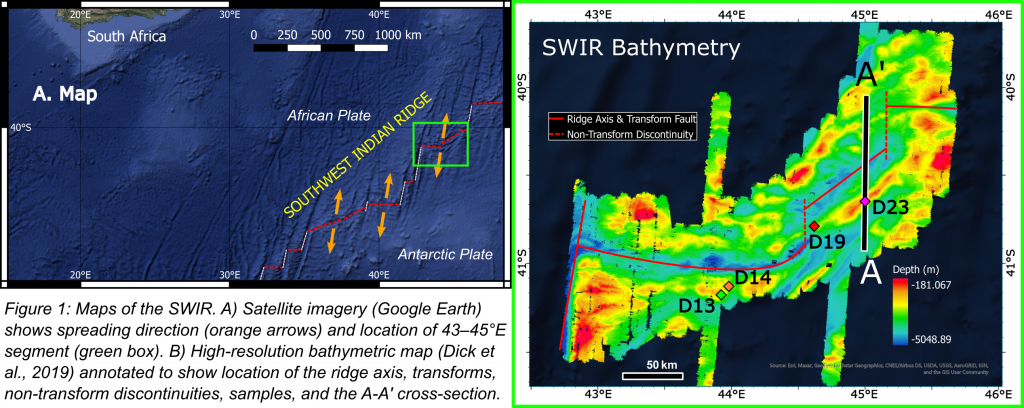
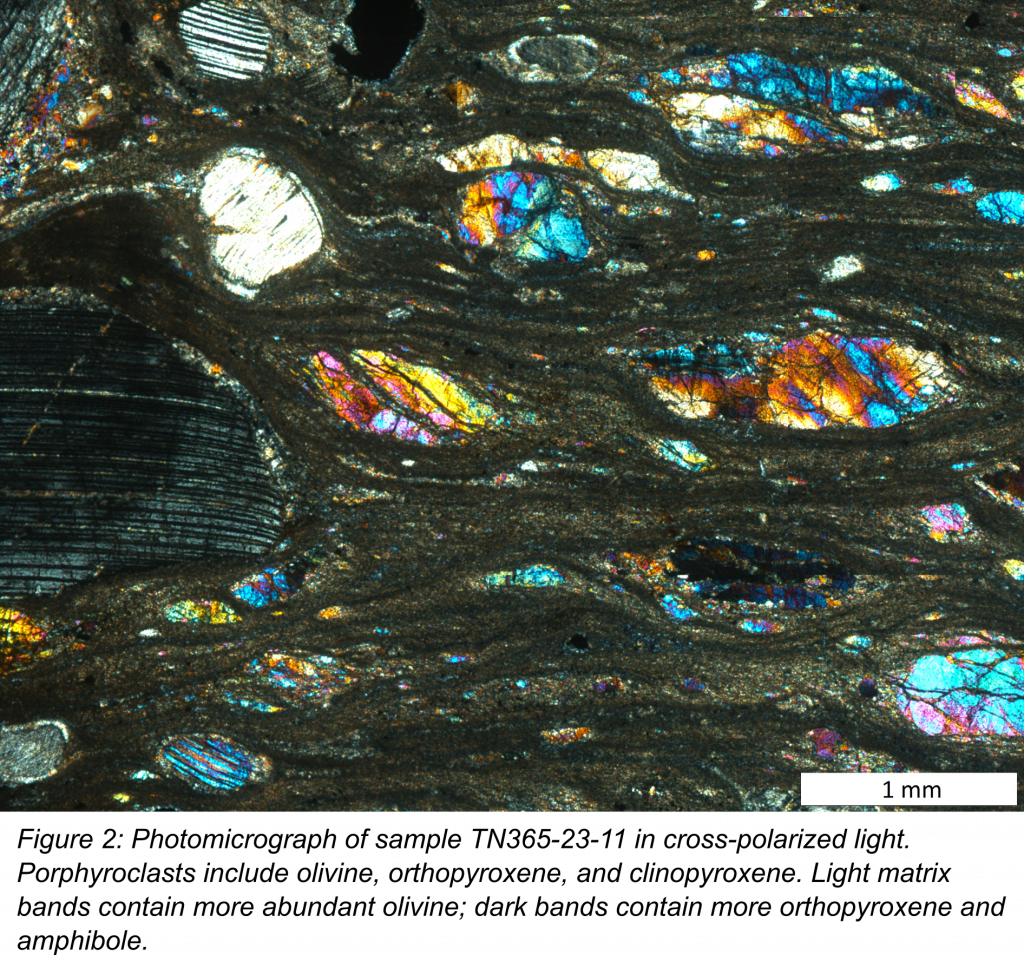
Petrography (Fig. 2), electron backscatter diffraction, and quantitative chemical analyses reveal a mylonitic matrix comprised of olivine, orthopyroxene, secondary amphibole, serpentine, and spinel-group minerals, with grain sizes of 3–8 µm. Crystallographic preferred orientations of matrix olivine show A, B, C, E, and AG slip systems, implying the olivine contained water (~700–2000 ppm H/Si) during deformation. Olivine deformation mechanism maps suggest plastic deformation initiated at ~1100 °C and ~29 km depth and ended at ~500 °C and ~13 km depth.
Weakly deformed pargasitic amphiboles are Na- and Cl-rich (Fig. 3), evidence of hydrothermal reactions with saltwater at up to ~875°C. The observed range of amphibole compositions and types-I, -III, and -IV deformation fabrics together reflect the down-temperature (~875–500°C) metamorphic history of these mylonites as they were hydrated by seawater and faulted to the surface.
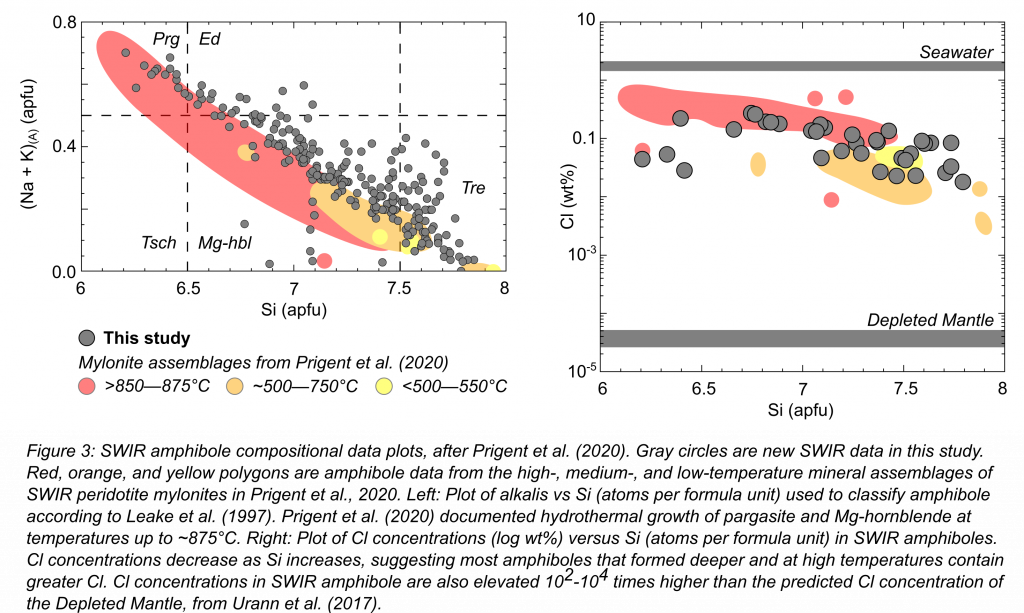
Although large-offset normal faulting is favored by reduced magmatism at slow spreading MORs in numerical models (Tucholke et al., 2008), the length of exposed footwalls measured from 60-m resolution bathymetry (Fig. 1) are less than or equal to the estimated ~13–20 km depth of the brittle-ductile transition, implying that multiple, cross-cutting faults are required to unroof peridotite mylonites to the surface (Fig. 4). The resulting highly fragmented lithosphere provides more conduits for hydrothermal circulation, and a linear temperature gradient predicts that the ingress of seawater may occur to depths of ~23 km below the SWIR, much deeper than previously thought. Although the importance of deeper hydrothermal circulation in geochemical cycles is unknown, these findings are essential for future studies of mineralization, ecosystems, and the geobiology of the seafloor.
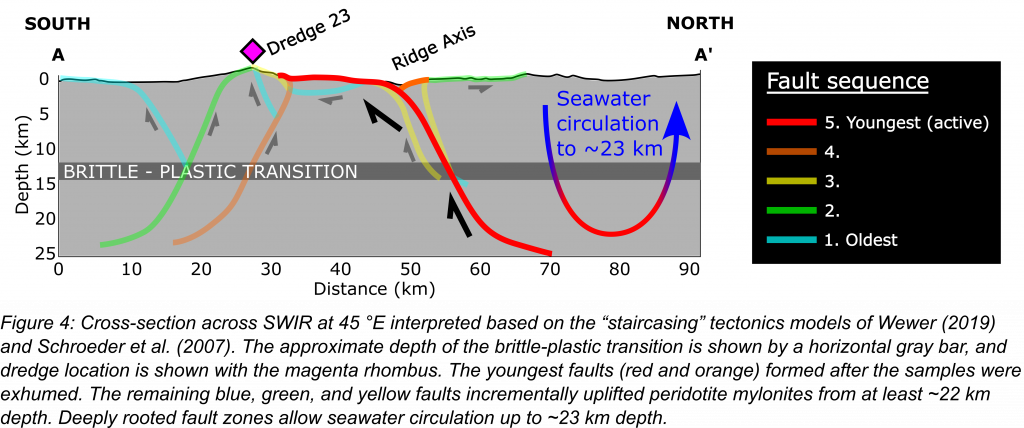
Presentations:
Colwell, D.J., 2023, Deep seawater incursion and high-temperature amphibole growth along cross-cutting detachment faults at the Southwest Indian Ridge: M.S. Thesis Defense, Department of Geology and Geophysics, University of Wyoming, Laramie, Wyoming, 8 March.
Colwell, D.J., Cheadle, M.J., John, B.E., Swapp, S.M., Brunelli, D., Dick, H.J.B., and Urann, B.M., 2021, Exhumation of mantle at the Southwest Indian Ridge: Constraints on temperature and depth of water ingress during detachment faulting: Abstract 102-9 presented at 2021 Fall Meeting, GSA Connects, Portland, Oregon, 10-13 October. doi:10.1130/abs/2021AM-370438
Thesis:
Colwell, D.J., 2023, Deep seawater incursion and high-temperature amphibole growth along cross-cutting detachment faults at the Southwest Indian Ridge [M.S. Thesis]: University of Wyoming.
2. Geological Map Series of the Phantom Lake Quadrangle in the Medicine Bow Mountains of southeastern Wyoming
State Map Project, Wyoming State Geological Survey (2021—2022)
Rachel Toner, Kelsey Kehoe, and Daniel Colwell
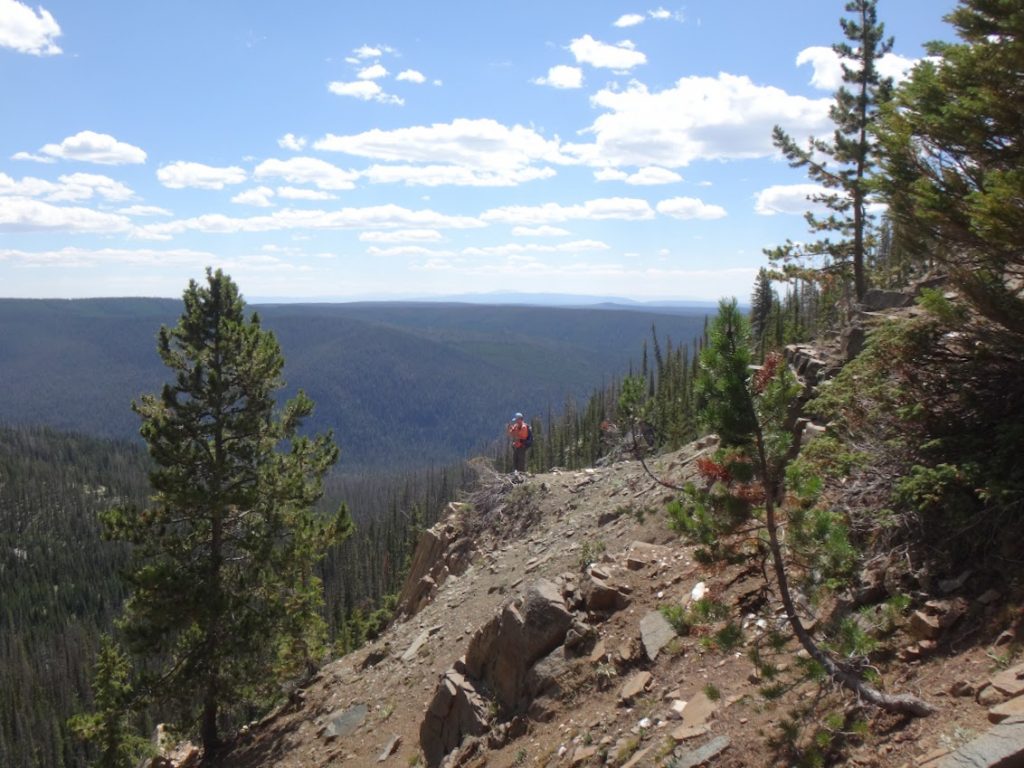
The Snowy Range of the Medicine Bow Mountains is a popular destination for locals and visitors to explore on foot, skis, snowmobile, or off-road vehicles, depending on the season. But, the area has also seen a boom of prospectors looking for gold and other resources in the past, and it has a rich geologic history dating back to the Late Archean, more than 2.5 billion years ago. The USGS and WSGS together have taken a greater interest in mapping this lesser explored mountain range at a small scale to look for mineralization associated with the Cheyenne Belt suture zone and to better understand the structural and geologic history.
Phantom Lake sits immediately west of Medicine Bow Peak at the Archean core of an anticline around which these mountains were built, and is just six miles northwest of the Cheyenne Belt. This shear zone, which cuts across the southeastern corner of the state, is the boundary where an island arc collided with the ancient rocks of the Wyoming craton around 1.7 billion years ago. Complex geological structures and widespread glacial deposits made mapping the geology of this area both challenging and exciting.
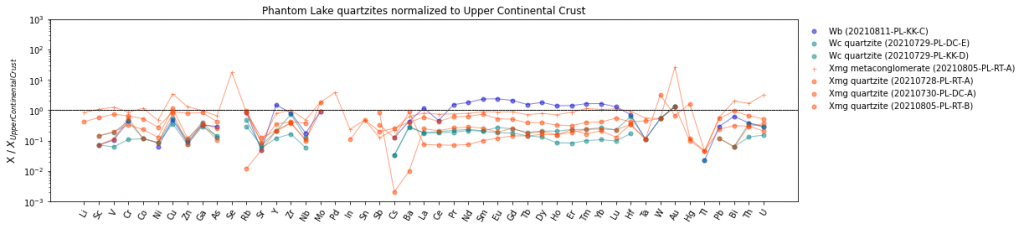
While mapping, I collected samples of every map unit for whole-rock geochemical analyses and generated array of various ternary and spider plots, such as the one above. Several different igneous, metamorphic, and sedimentary rock units were also selected for geochronological study to obtain more precise ages (see map). Surprisingly, we found that the red-pink granite is older than the quartzofeldspathic gneiss, which was previously thought to be the oldest unit in the Phantom Lake Quadrangle.
Download the map here.
3. 3D-modeling and magnetic (AMS) analysis of Mesozoic diabase dikes in North Carolina
Undergraduate Honors Thesis Research, University of North Carolina at Chapel Hill (2018 – 2019)
Daniel J. Colwell1, Allen F. Glazner1, William J. McCarthy2
1University of North Carolina at Chapel Hill, 2University of St Andrews
Diabase dikes that formed during Triassic-Jurassic breakup of Pangea are well-exposed in quarries in eastern North Carolina. In a quarry north of Rocky Mount, NC, steeply dipping, north-striking dikes up to 10 m thick cut ~300 Ma granite of the Rolesville batholith. We created 3D maps of dike outcrops and conducted anisotropy of magnetic susceptibility (AMS) analyses to determine the direction of magma flow.
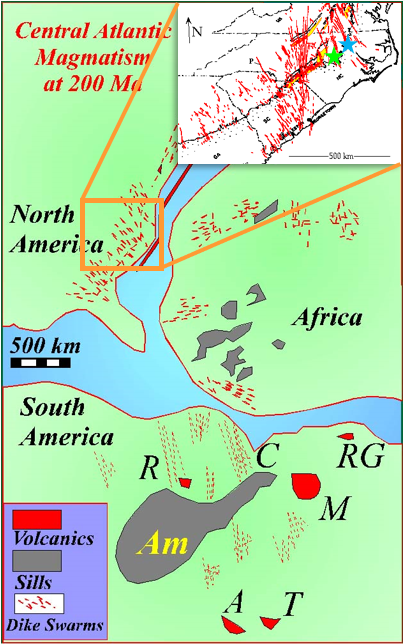
At the end of the Triassic period, basaltic lavas flowed over many parts of eastern North America, western Africa, South America, and Europe as Pangea broke up and the Atlantic Ocean formed. This event, which produced the Central Atlantic Magmatic Province (CAMP), ravaged ecosystems and likely contributed to the end Triassic extinction (Blackburn et al., 2013). Where did these lavas come from, and how did they travel through the crust?
The dikes through which these magmas flowed crop out extensively in eastern North Carolina, and are well exposed in quarries across the Piedmont . Here we present 3D reconstructions of these excavated dikes and estimates of the magma flow direction from magnetic analysis.
Left: Distribution of CAMP magmatism during the breakup of Pangea (Marzoli et al. 1999). Inset from Ragland et al. (1983) shows dike locations in the southeastern US. Blue star marks the Nash County Quarry in NC.
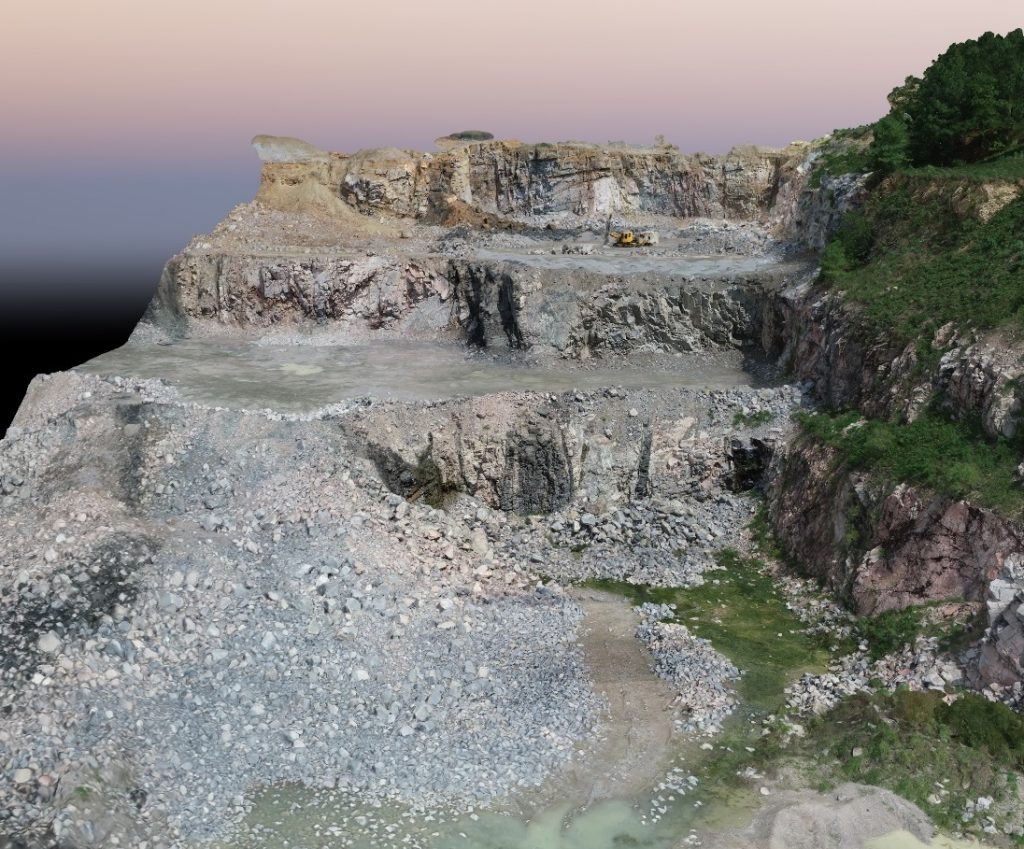
Excavation at the Nash County Quarry exposes dikes on each bench level, revealing changes in the structure and interconnectivity of the dikes both laterally and vertically. Continued excavation of the dikes allowed us to create a collection of 3D models of the same dikes across time, and we used these models to visualize more of the dikes’ 3D structure. At the outcrop scale, each 3-10 m thick dike is composed of multiple smaller dikelets 20-100 cm wide.
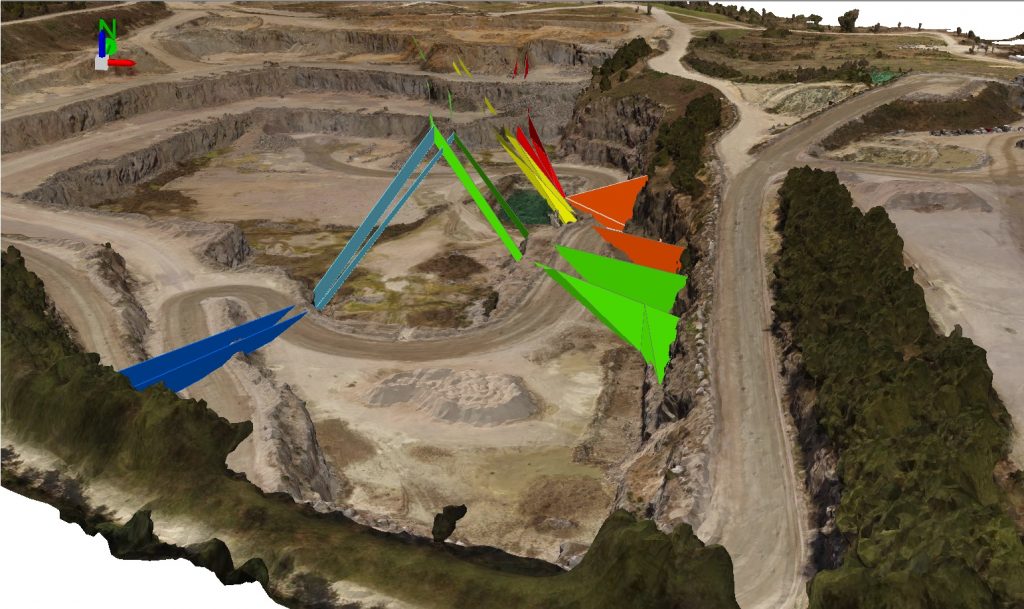
We conducted aerial surveys with ground control points and used Pix4D Mapper and Cloud software to construct high-resolution, georeferenced 3D models of each set of dike exposures. 3D polylines were traced along the contacts between the dike and granite wall rock, and these were combined with a 3D model of the whole quarry in ESRI ArcScene to visualize, measure, and reconstruct dike planes across the excavated area (left).
Magma flow tends to foliate or lineate crystals along the direction of flow as they form, resulting in a subtle mineral alignment in the fully crystallized rock. We used anisotropy of magnetic susceptibility (AMS) analysis on oriented diabase samples to measure the changing magnetic foliation orientation across the width of a dike. The figure on the right (Porreca et al., 2015) shows how these foliation measurements can be used to estimate the direction of magma flow.
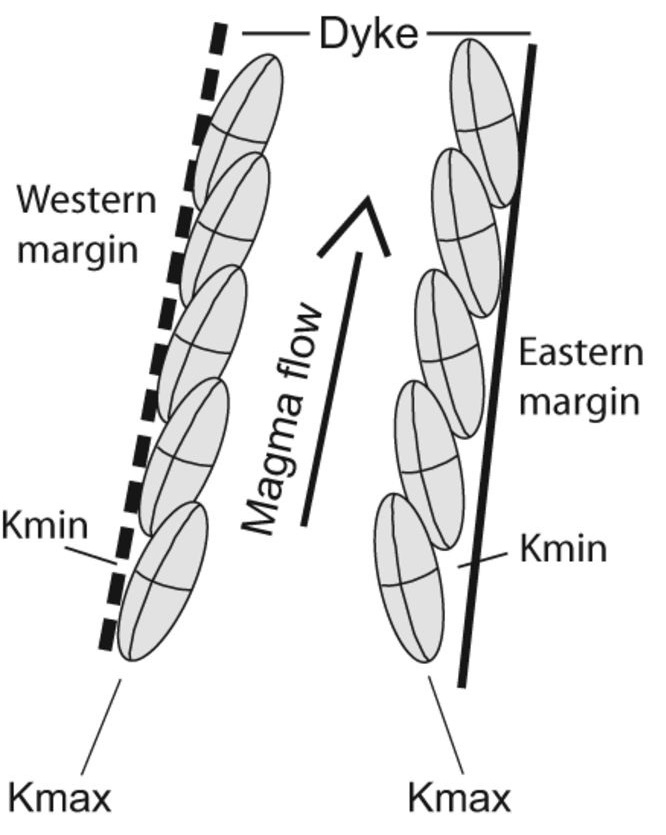
Magnetic foliation planes collected across multiple dike outcrops at the Nash County Quarry are plotted on the stereonet to the right. The foliation planes most frequently strike northeast or are imbricated on either side of the dike plane (yellow). This geometric relationship indicates horizontal magma flow, but more extensive sampling and AMS analysis is needed to constrain an azimuth of magma flow.
Ernst and Baragar (1992) conducted a much broader AMS study of the MacKenzie dike swarm in northwestern Canada, which radiates outward from a focal point on Victoria island, similar to the CAMP dikes. Magma in the MacKenzie dikes flowed vertically near Victoria Island, and more horizontally as it traveled away from the source. AMS results from the dikes in North Carolina suggest a similar pattern of outward horizontal flow from the paleo-center of this magmatic province.
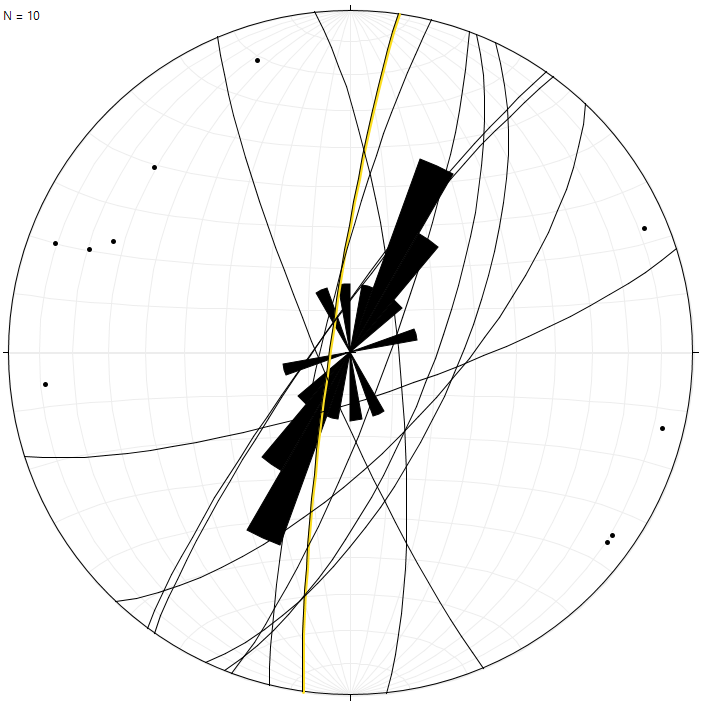
Presentations:
Colwell, D.J., Glazner, A.F., and McCarthy, W.J., 2019, 3D-modeling and magnetic (AMS) analysis of Mesozoic diabase dikes in North Carolina: Abstract 11-46 presented at 2019 Meeting, GSA Southeastern Section, Charleston, South Carolina, 28-29 March. doi:10.1130/abs/2019SE-327844
Colwell, D.J., Glazner, A.F., and McCarthy, W.J., 2019, 3D-modeling and magnetic (AMS) analysis of Mesozoic diabase dikes in North Carolina: Poster presented at 2019 Anadarko Student Research Symposium, Department of Geological Sciences, University of North Carolina at Chapel Hill, 12 April.
Thesis:
Colwell, D.J., 2019, 3D-modeling and magnetic (AMS) analysis of Mesozoic diabase dikes in North Carolina [B.S. Honors thesis]: University of North Carolina at Chapel Hill, 27 p.